Physics facts
Here you will learn about the amazing Quantum Physics behind the game.
What do you want to learn about?Dirac’s notation
If you’ve been wondering about the |↑> and |↓> symbols scattered all over the game, they are part of the bra-ket notation. Consider them like a shorthand code for describing an object using its attributes. Say you have an apple: its size, color, weight, flavor, and so on, are all parts of its description. We can use bra-ket notation to express its properties in order:
|size,color,weight,taste,other properties…>
In the quantum world, we use this notation to describe the state of particles like electrons, in which case we call it a quantum state!
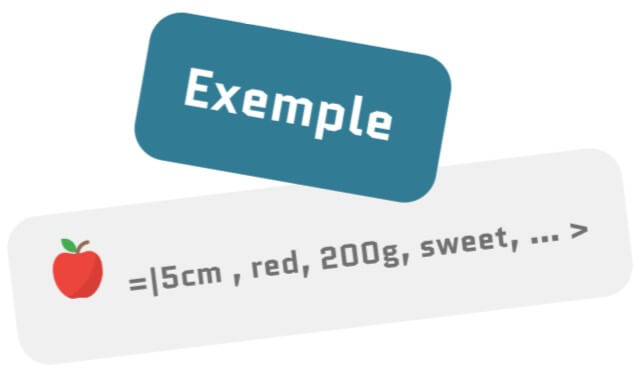
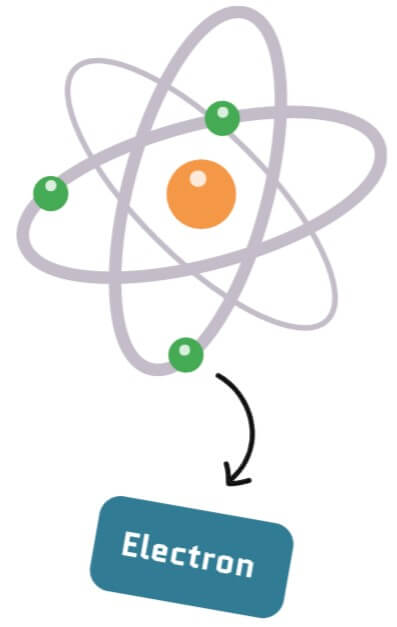
Electrons and spin
Electrons are infinitely small particles that can be found around an atom’s nucleus, kind of like mosquitos swirling around a lamp. They have properties like mass and charge (just like anything you can hold or touch) but also a unique property called spin.
The spin is a number that determines how many directions a particle can be oriented. The spin of an electron is 1/2, which means that it can always be found in two opposite directions which we call up and down, i.e. in a quantum state |↑> and |↓>.
Electrons belong to a larger family of particles called fermions, all of which follow the Pauli’s exclusion principle. It’s like in a conference – no two attendees (fermions) can occupy the same seat (quantum state) at the same time.
Superposition
The direction of the spin isn’t set in stone – it can change in unexpected ways! In quantum mechanics, electrons can be superposed, a state where the spin isn’t definitely up or down but somewhere in between. But once we measure it, the superposition collapses, and we find the spin is either up or down, as if the electron just made up its mind at the last moment.
Even more bizarre, two electrons can be entangled, meaning that they have a combined superposed state: when measuring one electron, the other collapses immediately, no matter how far it is!
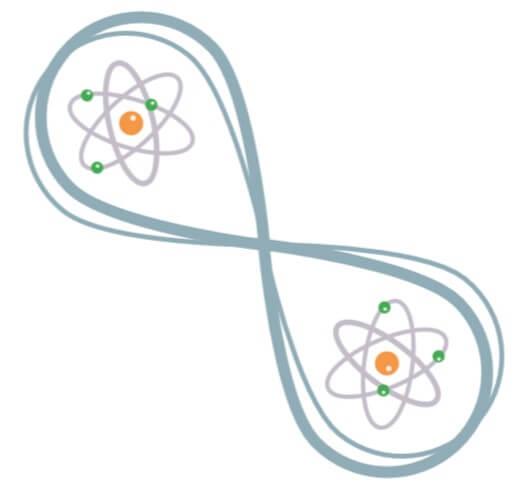
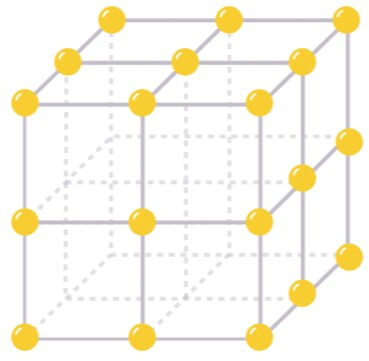
Lattice
Atoms in a material can be organized in grid structures called lattices – like houses on a street. Each lattice site can house up to two electrons with different spins, just like a two-bedroom house. Under some conditions, the movement of electrons in a lattice resembles a series of hopping events from one atom to another. This is called the tight-binding approximation.
Electrodes
Imagine you’re trying to make some hikers (electric current) move through a trail (a lattice). You would need to usher people in at one end (the source) while making sure they can leave from the other end (the drain). In our game, we only have sources. So, electrons flow from one source to another, causing a short-circuit.
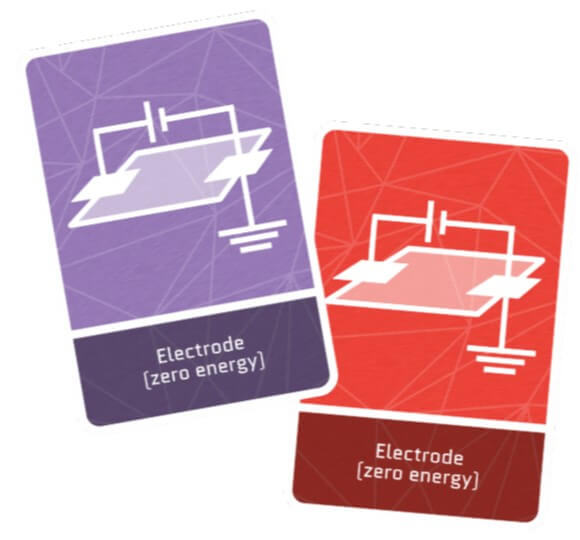
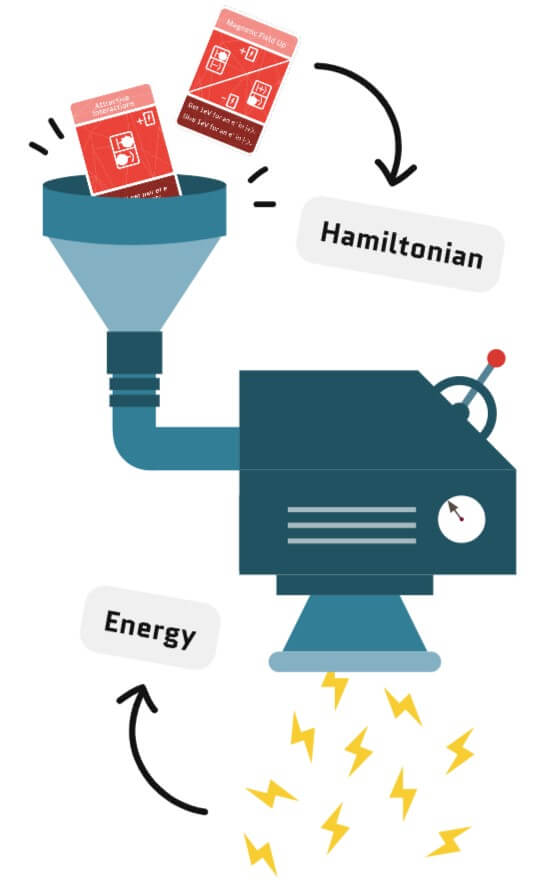
Energy conservation is a key principle in quantum physics, which means the total energy of the electrons on the lattice cannot change when the electrons move. The Hamiltonian is like an energy calculator – it tells you how much energy a configuration of electrons has based on two factors: the orientation of their spins (magnetic field) and how many electrons share a site (interactions).
Magnetic field
Without a magnetic field, the spin of an electron doesn’t affect its energy. But when you add a magnetic field, you get a Zeeman splitting, where one spin direction becomes more energetic than the other.
Interactions
Electrons can either repel or attract each other, which changes their total energy.
Usually, electrons repel each other, so squeezing two into the same site costs extra energy. But in some cases, electrons can attract each other, making it energetically favorable for them to pair up. In the game, this means releasing energy from the board to the player.
Total energy
The sum of the two terms gives the total energy of the electrons in a site. Using our interactive tool, you can configure each term independently and see how the energy of each configuration changes.
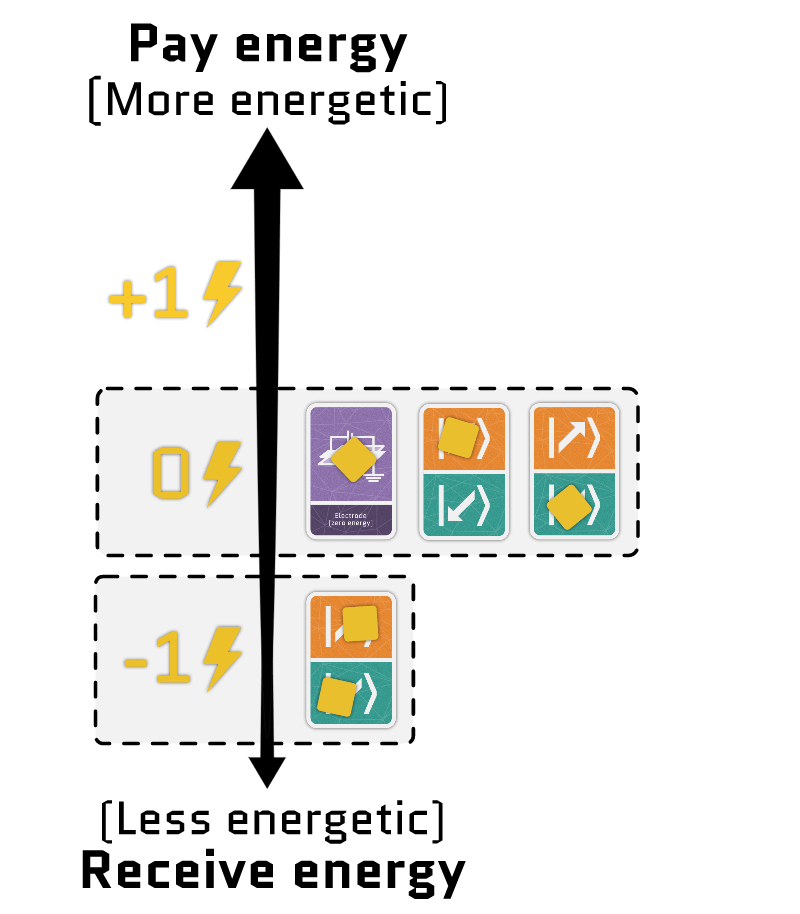
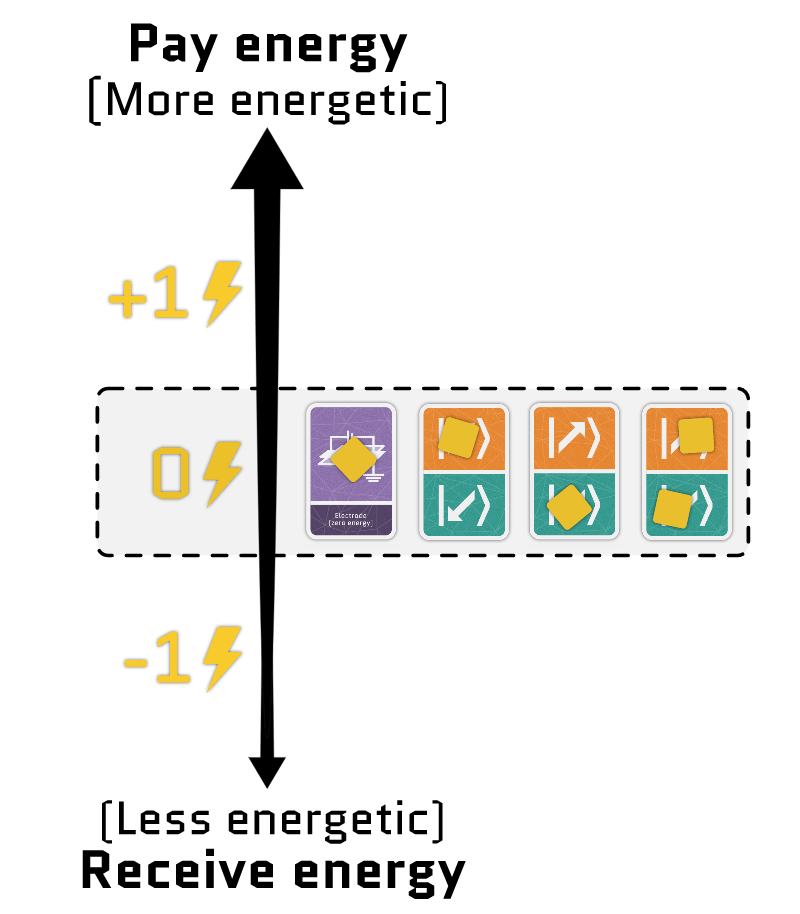
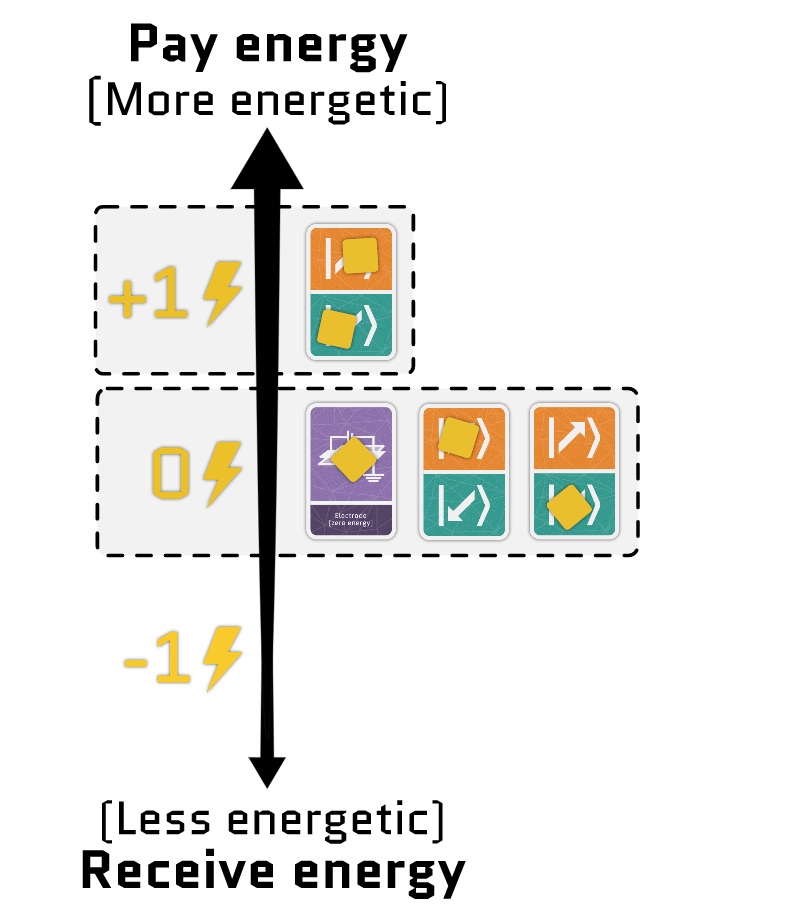
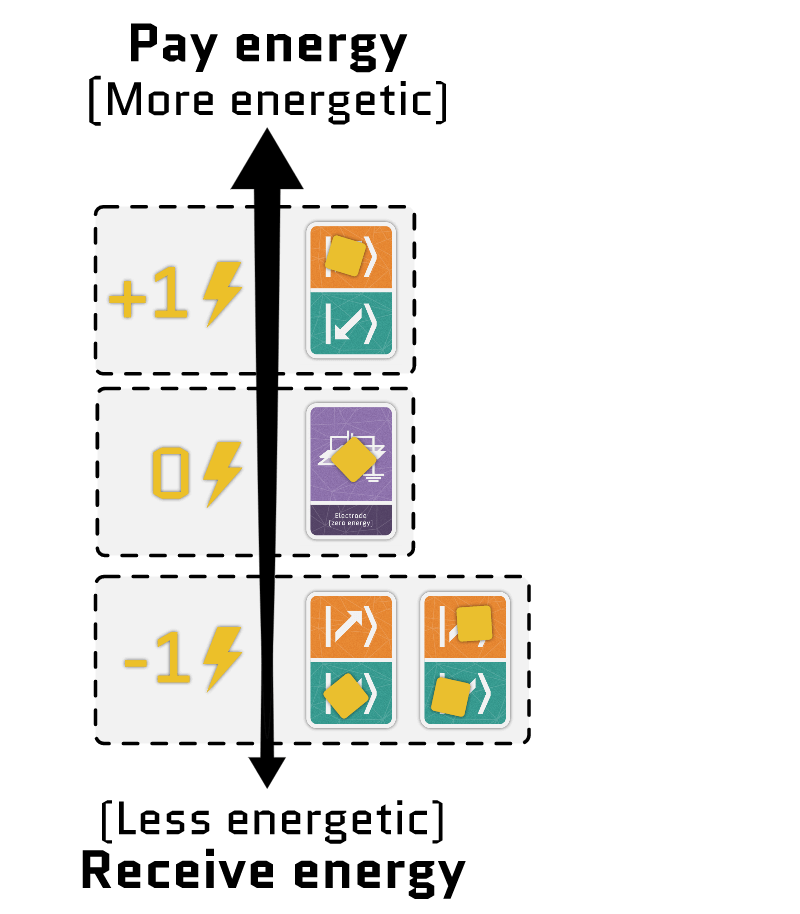
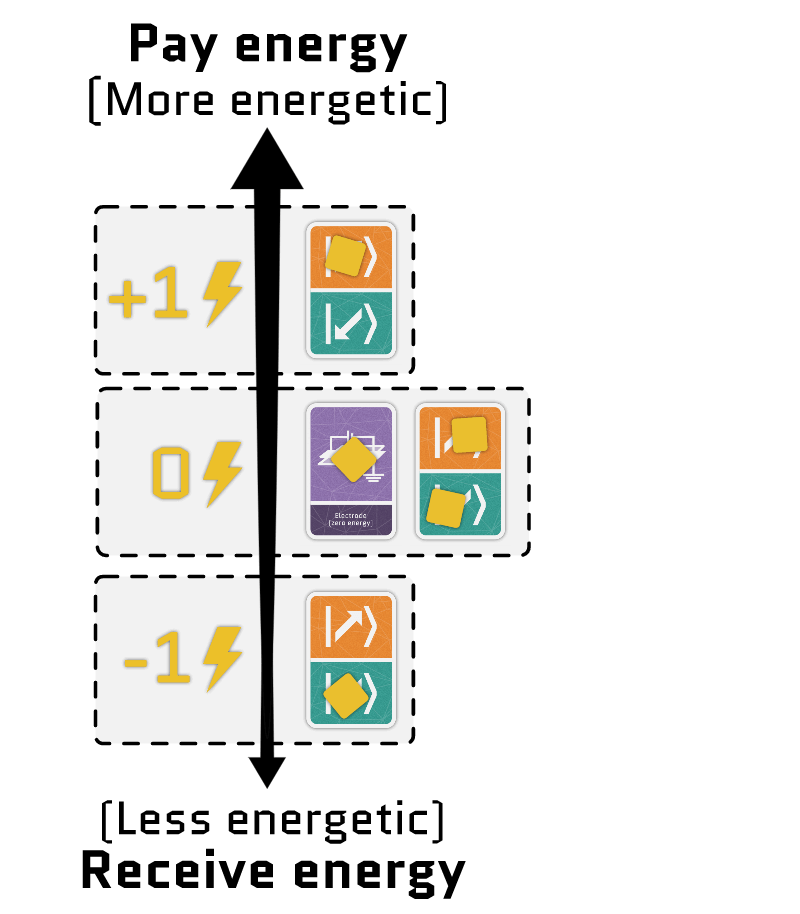
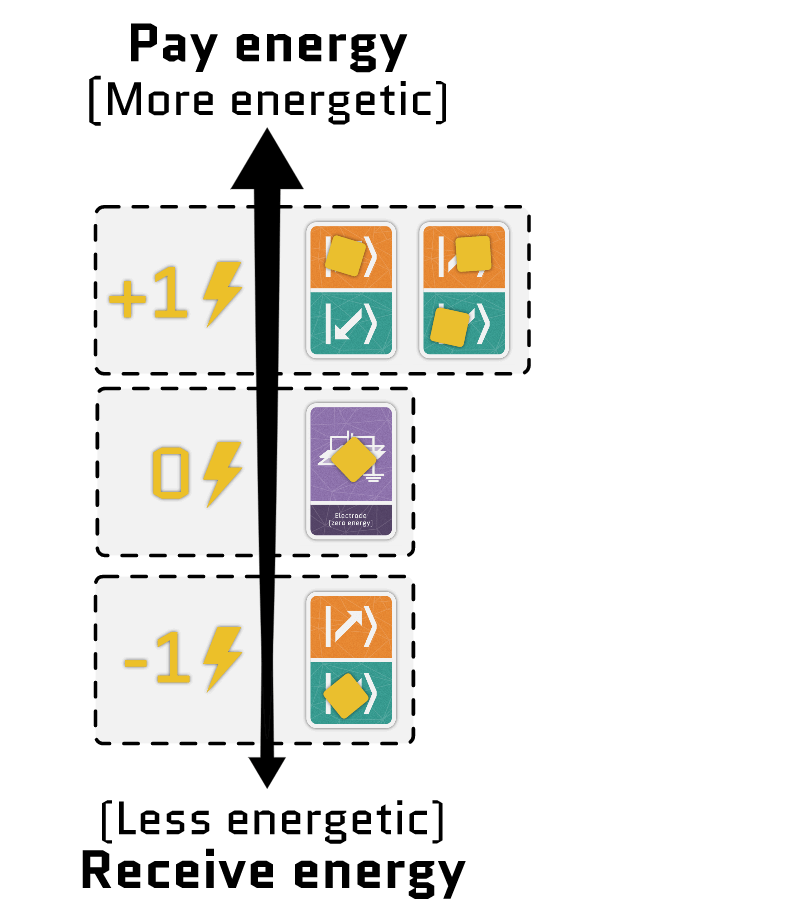
Movement cards
Hopping
Picture an electron moving within a material made of atoms. If the nucleus of an atom pulls the electron too strongly, it doesn’t just glide smoothly; instead, it hops from one atom to another, kind of like a frog jumping from lily pad to lily pad. But the farther the lily pad, the harder it is to reach.
Doublon Hopping
Now imagine two electrons finding themselves at the same site, despite them naturally trying to keep their distance due to their charges. This phenomenon occurs in some unique materials and results in a doublon. It’s as if these two electrons are buddies who stick together despite the crowd trying to pull them apart.
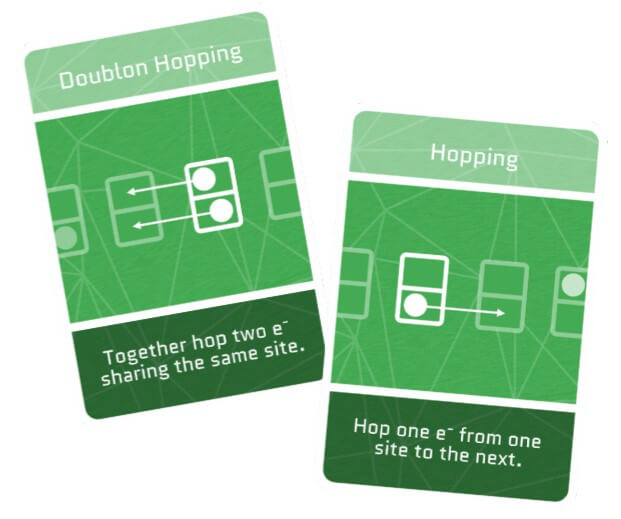
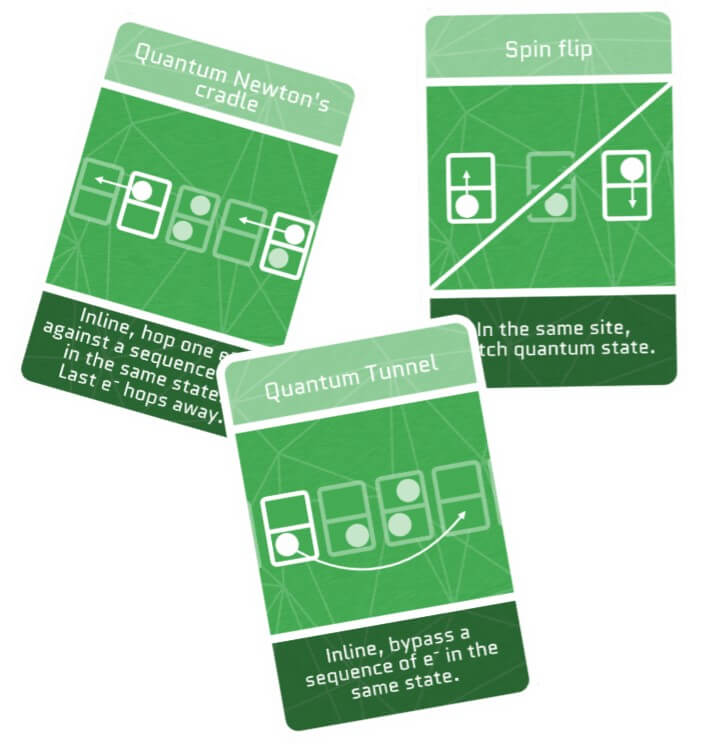
Spin-flip
The spin of an electron interacts with the surrounding electromagnetic fields in various ways. One of these interactions is called spin-orbit, which allows electrons to exchange their spin. It’s like swapping the sports team mid-season!
Quantum Tunnel
Quantum particles have a magical ability – they can pop up anywhere in the universe! This is because their position isn’t rigidly fixed. This trick allows them to tunnel through areas they’re not supposed to access, like a ghost walking through walls. The odds of this happening depend on the size of the no-go area. The larger the area the more unlikely it is!
Quantum Newton’s Cradle
Do you remember the Newton’s cradle, the toy on your desk with swinging steel balls? Just like those balls, quantum particles sometimes can also pass their energy and momentum when colliding, bouncing back and forth without losing energy.
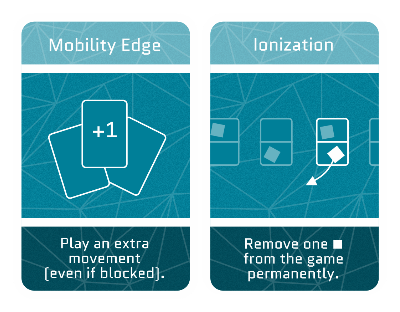
Ionization
Each electron in an atom has a region where it spends most of its time, called an orbital. When an electron absorbs light, it can switch orbitals. But if the light is too energetic, the electron gets kicked out of the atom altogether!
Mobility Edge
Sometimes, if there’s not enough energy around, electrons might get stuck and can’t move. To get them moving again, we need to give them a little energy boost. This switch from being stuck to moving again is called a mobility edge, like pushing a stalled car to get it rolling.
Resistance
Resistance is why the filaments in old lamps glow when you turn them on. The electricity (a stream of electrons) bumps into stuff in the lamp filament, and each collision turns some electrical energy into heat, causing the filament to glow.
Heat source
Electrons can absorb energy when in contact with a hot body, effectively cooling it down in the process. If the body is so large that the temperature change is negligible, it is called a heat source.
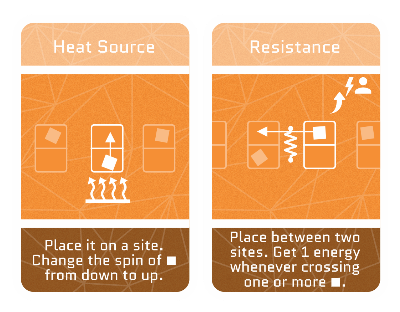
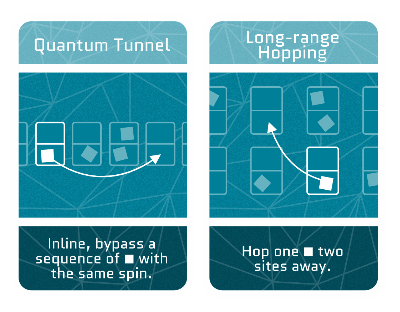
Quantum Tunnel
Quantum particles have a magical ability – they can pop up anywhere in the universe! This is because their position isn’t rigidly fixed. This trick allows them to tunnel through areas they’re not supposed to access, like a ghost walking through walls. The odds of this happening depend on the size of the no-go area. The larger the area the more unlikely it is!
Long-range hopping
Imagine a tiny frog computing all the jumping possibilities. Most likely he will make it to the nearest lilypad but the chances of getting to the next-to-nearest target are never zero. Electrons can sometimes also hop to atoms far away.
Impurities & vacancies
Atoms in a material ressemble neatly arranged apples on a supermarket shelf. But sometimes, there’s a gap where an atom is missing, called a vacancy, or an extra atom sneaks in between, called an impurity.
Penning trap
Penning Trap: Just as a bobblehead toy’s head is held in place by a spring, electrons can be trapped in place by electromagnetism. In a Penning trap, when an electron attempts to escape, it encounters an electric field that pushes it back in place.
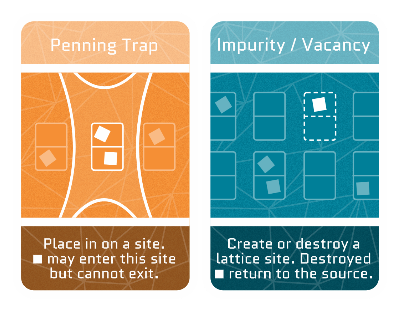
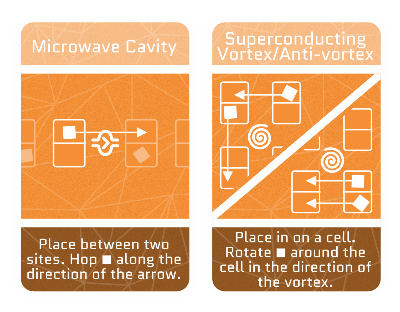
Microwave cavity
Because electrons are charged particles, they like to move along the direction of an electric field. In a cavity, the electric field is perfectly aligned to shoot out any incoming electrons, like cannonballs!
Quantum vortices
Superconductivity is like the superhero state of matter, where, among other things, electrons don’t lose energy while moving around. When we run a magnetic field through a superconducting material, electrons whirl around certain points, forming vortices, just like how leaves whirl around a tornado’s center.
Diffusion
When electrons move, they often bump into other electrons or atoms. This makes them slow down and scatter more, like a crowd dispersing at the end of a concert. This effect, known as diffusion, is why electrons don’t always go straight from point A to point B.
Superposition
See the Superposition section above.
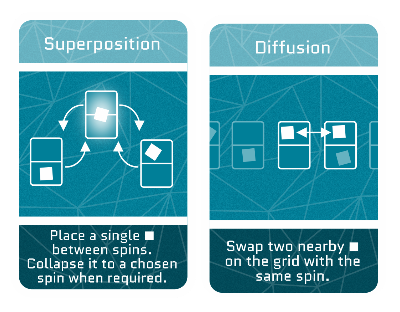
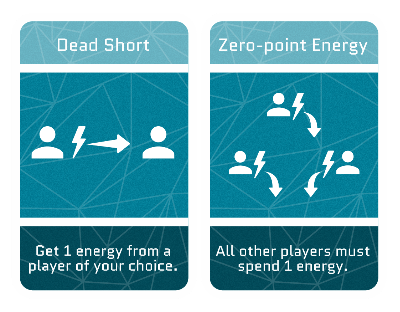
Zero-point energy
It is not possible to completely remove the energy of a quantum system. Even if you cool the system down to its absolute minimum, it will still retain its finite zero-point energy (often in the form of vibrations).
Dead short
Electrons always travel through the path of least resistance. If we accidentally connect two electrodes without any resistance in between, all the electrons will flow through them, heating up the system and causing a dead short.